We already met our
Milky Way galaxy in the
Our
Galaxy section. We also discussed
stars which are
members of
galaxies. Most of the beautiful
phenomenon we see in the
night sky -
comets,
planets,
star clusters,
planetary nebula and diffuse
nebula - are all around or within our own
galaxy.
Beyond our
galaxy are other interesting phenomenon:
By studying these
galaxies and their motion
relative to us and each other we learn about how our
Universe was formed. What we know about
galaxies gives vital clues as to how the Universe began.
It has been recently discovered that while there are a few spiral galaxies in the early Universe, the majority of galaxies are elliptical. As for the size, they are surprisingly small compared to our own galaxy.
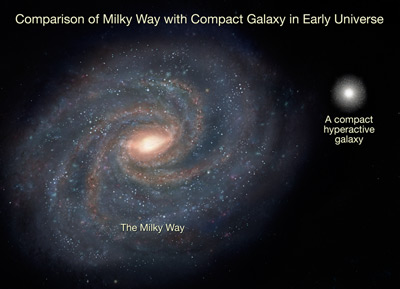
There is a variety of galaxies, all with
different sizes and shapes. The work of Edwin Hubble
allows Astronomers to categorize the variety of
galaxies on a diagram called the Tuning Fork
diagram, or the Hubble Tuning Fork diagram. While
initially thought of as an evolutionary diagram for
galaxies, we now know that most galaxies do fit in a
defined category:
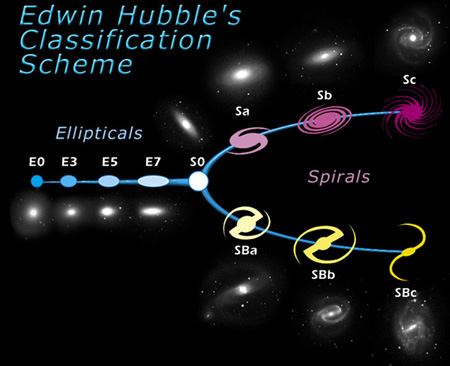
(Image
Credit)
Visit the
Galaxy Morphology page for a real world example
of analyzing galaxy types.
There are basically two major types
of galaxies:
-
Elliptical galaxies
-
Spiral galaxies
Elliptical galaxies are unique in
that there is no organize structure of stars. There
are no spiral arms and stars travel in just about
any direction. Giant elliptical galaxies are very
rare and can be 20 times the size of any other
galaxy. More common are dwarf elliptical galaxies
which are smaller than spiral galaxies and contains
only a few million stars.
Spiral galaxies are more organized,
containing spiral arms that appear orderly in
motion. The average number of stars in a spiral
galaxy is about 100 billion (billion with a b)!
Spiral galaxies themselves have three major
categories:
-
Normal spirals
-
Barred spirals
-
Irregular
A normal spiral galaxy have their
arms connected directly to the core of the galaxy
while barred spirals have their arms connected to a
protrusion (called a bar) emanating from the core.
The grading scheme of the Tuning
Fork is as follows:
-
E0 is a round elliptical galaxy
-
E7 is a near disk
-
S0 can look like an elliptical
galaxy, has a more thinned disk
-
Sa is a normal spiral with a
bright central bulge and a tight spiral
structure
-
Sc is a normal spiral with a dim
bulge and a loose spiral structure
-
SBa is a barred spiral with a
bright central bulge and a tight spiral
structure
-
SBc is a barred spiral with a
dim bulge and a loose spiral structure
The numbers and letters in between
(i.e. E1, E2, Sb, Sb1, SBb, SBb1, ect.) are all
based on visual interpretation.
Irregular galaxies are a group
recently added to the Tuning Fork diagram (though
not seen above). Irregular galaxies have some hints
of organized spiral structures, but are loosely
scattered.
A galactic comparison:
|
Absolute Blue Magnitude: |
Mass (Solar Mass): |
Disk Diameter (kiloparsecs): |
Elliptical
Galaxies: |
-8 to -23 |
107 to 1013 |
~0.3 to 100 |
Spiral Galaxies: |
-16 to -23 |
109 to 1012 |
5 to 100 |
Irregular Galaxies: |
-13 to -20 |
108 to 1010 |
1 to 10 |
Here are some examples of a variety
of galaxy types:
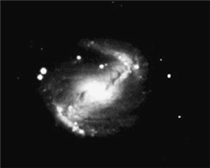 |
 |
SBb - Galaxy NGC5383 |
E0 - Galaxy
M87 |
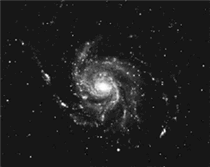 |
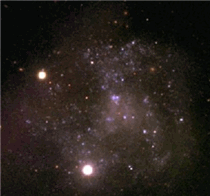 |
Sc - Galaxy
M101 |
Irr - Galaxy IC4182 |
Active Galactic Nuclei is a term that describes
four types of galaxies:
Active Galactic Nuclei, or AGN,
produce bright emissions of non-thermal
synchrotron
radiation by a common source - a supermassive
black
hole. It is believed that every galaxy has at its
core a supermassive
black
hole. This includes our
galaxy. AGN's exist when the black hole has material
to "feed." This material can be stellar debris,
molecular clouds of hydrogen, even stars. If the
black hole is large enough, it can strip nearby
stars of their atmospheres. More information on
black
holes can be found in the
Stars section.
Synchrotron radiation is the source
of jets and lobes that are seen with AGN's. Magnetic
field lines created by the supermassive
black
hole
traps electrons from ionized particles near the
accretion disk. These electrons are sent out in jets
at almost the speed of light - these are called
"relativistic jets." Note: the term
"relativistic" is used for any phenomenon traveling
near the speed of light.
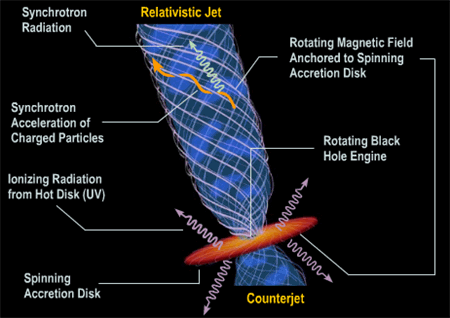
(Image credit: Brooks/Cole Thomson Learning)
One of the major differences between
normal galaxies and AGN's is their measured
spectra. Non-thermal emission is detected by
spectrometer and is responsible for the
extended spectra seen here in the example on
the right.
A normal galaxy has the characteristic
bump seen on the lower part of the image
while the AGN's have dominating non-thermal
emission primarily from
synchrotron
radiation. |
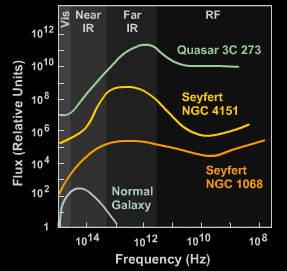
(Image credit: Brooks/Cole Thomson Learning) |
Radio galaxies are giant elliptical
galaxies that look normal when viewed through a
telescope, but emit very strong radio emissions.
While using an optical telescope, a radio galaxy
will look like any other elliptical galaxy. But when
an astronomer uses a radio dish to examine a radio
galaxy, its "brightness" increases to nearly 1033
watts. The majority of
radio emission is in the form
of radio lobes, although a halo around the core of
the galaxy can also emit
radio waves.
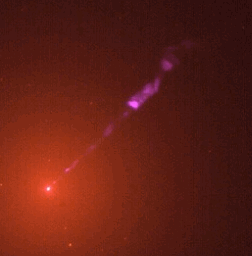 |
The image on the left is of elliptical
galaxy M87. Using the Hubble Space Telescope
and using an infra-red and near UV filters,
the core of the galaxy is visible as is the
emanating jet of
synchrotron
radiation. The
jet is moving rapidly, and in many cases can
travel near the speed of light. Such speeds
are often referred to a "relativistic."
When using
radio to examine a
radio galaxy,
the picture changes somewhat. |
 |
This image of Centaurus A has been
overlaid with its composite
radio image. The
radio lobes (shown perpendicular to the dust
lane colored in blue, green and red) are not
visible optically but are clearly visible in
the
radio spectrum. It is common for these
lobes to extend far out from the core. The
source of these lobes are the same as the
jets -
synchrotron
radiation. |
Seyfert galaxies
are considered radio quiet AGN's. They generally do
not emit strong
radio waves although few have been
seen with very small
radio lobes. Seyfert galaxies
are characterized by their extended non-thermal
emission lines as well as a predominantly bright
nucleus.
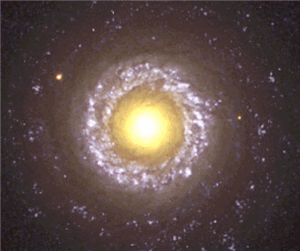 |
This image of NGC7742 is a typical
Seyfert galaxy. The nucleus - or center of
the galaxy - is often very bright, sometimes
10 times that of a normal galaxy.
In addition to this, Seyferts also emit
strong X-ray and IR emissions as well. This
indicates the engine of a Seyfert being a
supermassive
black
hole. |
Seyfert galaxies themselves are in two
categories:
- Seyfert 1 - both broad and narrow emission
lines, strong UV and X-ray emission
- Seyfert 2 - narrow emission lines and weak
UV and X-ray emissions but strong IR emission
The main reason behind Seyfert galaxies lack of
radio emission is though to be due to a smaller
"engine" (or a supermassive
black
hole that is not
as big as the ones inhabiting radio galaxies) and
large clouds of dust near the nucleus that absorb
higher energy photons and re-emit them at longer, IR
(InfraRed) wavelengths.
Both
radio and Seyfert galaxies appear to bridge
the gap between normal galaxies and quasars. This
has the suggestion that all galaxies go through and
evolutionary process that start out as quasars and
end up as a normal galaxy.
BL Lac objects are
named after the star BL Lacertae. Discovered in
1929, this star was believed to be a
variable star
(since the cores of AGN's do fluctuate in
brightness), but measurements of redshift place this
object at a distance equal to known distant
galaxies.
BL Lac objects emit very strong
synchrotron
radiation that suggests that the orientation of this
particular AGN is such that the
radio lobes and jets
are aimed toward us. As a result, the intensity of
the energy generated by the core is such that any
spiral structures are difficult to discern. A
characteristic of these objects are no emission
lines (or very weak ones) but are very strong
emitters of X-ray and IR wavelengths. As such, a BL
Lac object can look like a bright
variable star.
While quasars (covered below) are
radio loud, blazars - which have the same characteristics as a
quasar - are optically bright. A blazer is basically
a BL Lac object that has a high
redshift (meaning
its far away).
Quasars were discovered in
1963. These objects were believed to be stars that
emitted strong
radio waves - hence the name QUAsiStellAR objects.
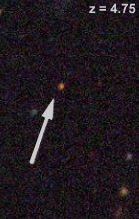 |
When viewed optically, a quasar looks
like a distant red star. The image on the
right indicates a quasar with a
redshift of
4.75 (which is about 1.7 x 1010
light-years away). One of the
characteristics of a quasar is an unusually
strong UV emission. While it is believed
that the major characteristic of a quasar is
its strong emission of radio waves, in
reality only 10% of all known quasars do
emit strong
radio. It is not clear as to why
this is. It is suggested that "radio quiet"
quasars lack the jets emanating from the
core. |
Instead, all quasars have in common strong
emissions of near-UV and near-IR wavelengths - the
result of which is primarily blue emission.
So if quasars are mostly blue, why do they look
red? The perceived red color of a quasar is a result
of its rapid
redshift. Quasars are being accelerated
by the expanding Universe and a very high rate of
speed, lengthen the wavelength as it travels back
towards our telescopes. In addition, any
interstellar dust will absorb all wavelengths and
re-emit them in red - this is called interstellar
reddening.
The Unified AGN Model:
As mentioned earlier, the primary
engine for AGN's is a supermassive
black
hole. The
variability in orientation of the back hole's
accretion disk and
radio lobes will indicate the
type of AGN. For example, a BL Lac object is an AGN
with its lobes pointed directly towards
Earth.
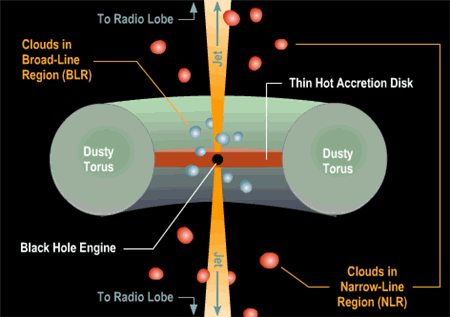
(Image credit: Brooks/Cole Thomson Learning)
Probably the most amazing thing
about the AGN is its size. The image above
illustrates the AGN portion of a galaxy, and the
diameter from dusty torus to dusty torus is less
than the diameter of our
Solar System! The types of radiation seen depends
on the orientation of the AGN. The emitted particles
vary depending on the source location and path of
emission:
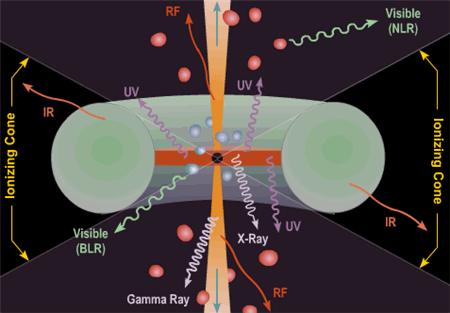
(Image credit: Brooks/Cole Thomson Learning)
So what do we see based on
orientation?
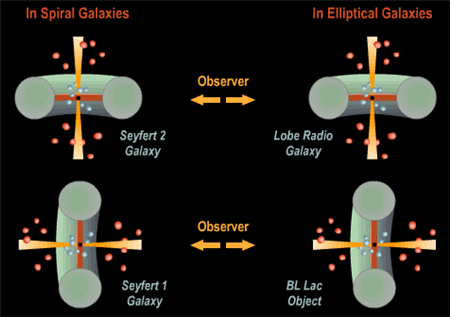
(Image credit: Brooks/Cole Thomson Learning)
It is important to realize that the
entire premise of AGN's lie in our collected
evidence of black holes. While no
black
hole has has ever
been directly observed, what we have seen so far
with AGN's is that the model fits observed data and
that strongly suggests that supermassive
black
holes
exist.
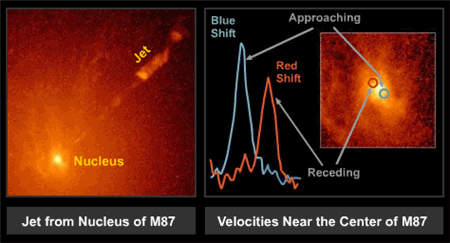
(Image credit: Brooks/Cole Thomson Learning)
The image above is one such piece of
evidence. Strong red and blue shifts of the bright
core of M87 suggests a rotating supermassive
black
hole - this visual evidence supports the AGN model
of the accretion disk with the expulsion of
synchrotron
radiation in the form of a jet.
Summary of AGN's:
Property: |
Quasars: |
Seyfert Galaxies: |
Radio Galaxies: |
BL Lac Objects: |
Galaxy Type: |
Spiral and Elliptical |
Spiral |
Giant Elliptical |
Elliptical |
Appearance: |
Compact, Blue |
Compact Bright Nucleus |
Elliptical |
Bright, Star-like |
Maximum Luminosity: |
100-1000x Milky Way |
Comparable to Bright Spirals |
Strong Radio |
10,000x Milky Way |
Continuum Spectrum: |
Non-thermal |
Non-thermal |
Non-thermal |
Non-thermal |
Emission Spectrum: |
Broad and Narrow |
Broad and Narrow |
Rare, Broad and Narrow |
Very Weak |
Absorption Lines: |
Yes |
None |
Yes |
None |
Variability: |
Days to Weeks |
Days to Weeks |
Days |
Hours |
Emits Radio: |
Some |
Weak |
Strong |
Weak |
Redshifts: |
z>0.5 |
z~0.05 |
z<0.05 |
z~0.1 |
Jets: |
Some |
Some |
Often |
Possible |
Back to Top |